Mycoforestry, For Ecological And Climatic Resilience
- Logan Keister
- Feb 21, 2021
- 25 min read
Logan Keister
2/5/2019
Mycoforestry, For Ecological And Climatic Resilience
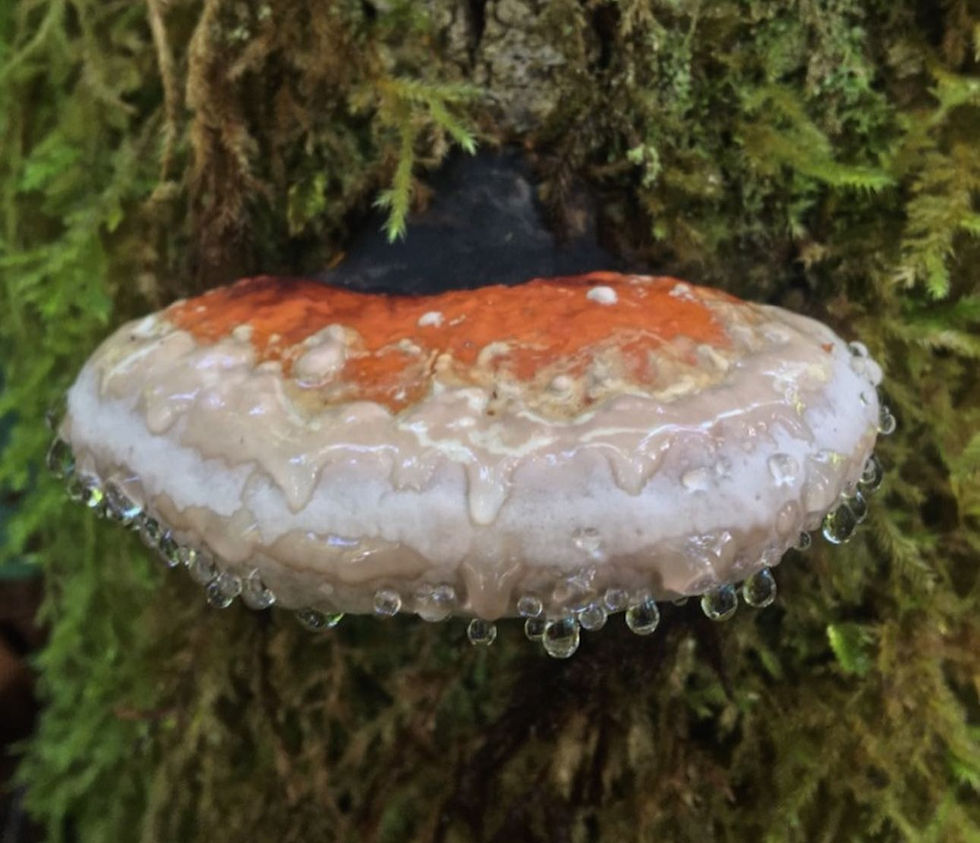
Fomitopsis pinicola (Red Ring Conk)
Introduction
Living in an era of uncertainty where materialism seems to dominate the drive of the human psyche, we are now facing the consequences of that drive. We all must extract some resources from our environment in order to survive, but we have come to a point where much of our extraction cannot be justified by the need for survival and often not even for improving quality of life. Today we are seeing trends of global increases in temperature, increasing prevalence of drought, depletion of groundwater reserves, loss of biodiversity, drastic changes in climate and increases in severe weather patterns (Fu et al., 2015). Most agree that these trends are largely driven by human industrialization and extraction (Goodess et al., 1992). Though many look to reduce carbon dioxide and greenhouse gas emissions through technological advances by increasing efficiency, it still seems that even going “carbon neutral” and reducing carbon dioxide emissions may slow down our current progression towards climate chaos, but will not stop it (Murray et al., 2009).
When looking for solutions on how to respond to these growing concerns, reforestation seems to be our most potent tool for combating climate change. Reforestation can be used to combat climate change since I and many others in the scientific community propose that deforestation is the main driver of climate change (Gorte et al., 2010). We have lost roughly 46% of the trees on this planet since humans first began harvesting trees (Nunez, 2019). With an uncertain future looming before us, we must do all we can to breed climate resilience and I see reforestation as our best strategy for reversing the effects of climate change. Not only for the ability of forests to store carbon but mainly for their ability to maintain our terrestrial hydrologic cycles which regulate our weather patterns.
Climate change has already presented us with many challenges towards our reforestation efforts. They found that in dry western U.S. forests that reforestation projects were often impeded due to increases in drought stress and increases in the frequency and severity of forest fires (North et al., 2019). These effects have caused increases in replanted seedling and sapling mortality rates. Increases in drought have been shown to increase the susceptibility of both young and mature trees to pathogens (Desprez et al.,2006). Human activity has brought in many “invasive” plants that hinder reforestation efforts from their increased competition with seedlings, and their greater ability to adapt to disturbances to the soil and adapt to changes in climate (Grove et al., 2019). Herbicides and other pesticides are often used on large swathes of land in reforestation projects to decrease pest and weed pressures on planted seedlings and saplings. These pest management practices increase uses of energy, fossil fuels and are detrimental to increasing forestry resilience due to nontargeted species mortality, reducing biodiversity (Pimentel, 1971).
The question is, how can we ensure that our reforestation efforts will breed resilient forests that survive future climatic changes, remain resilient in the face of ever-changing pathogen pressures, better utilize nutrients, efficiently biofiltrate pollutants, store carbon and maintain healthy terrestrial hydrologic cycles? The answers to these questions will not likely come from technological advances in reducing carbon output but will come from the forests themselves, and the symbionts that have evolved alongside our plants and trees for billions of years. Though the roles of all beneficial soil microorganisms deserve our attention, from looking at much of the research that has come about since the 1950s when it was first proven that fungi engage in mutually beneficial symbiotic relationships with trees (Melin & Nilsson, 1950), it seems to me that these symbiotic fungal species may be one of our greatest allies when it comes to ensuring that our reforestation efforts breed resilience in the face of climate change.
The first step to working with our fungal allies to help aid us in our reforestation efforts is to have a greater understanding of their ecological roles. Looking underground, we find that the ecology of our soil is deeply interwoven with an interconnected matrix of fungal hyphae collectively called mycelium. These mycelial networks under the soil create a matrix of connection between the majority of plant and tree species within our forest ecosystems. The symbiotic nature of fungi has long been ignored by science until recently and fungi found living in association with plant roots were long considered to be parasitic. It wasn’t until the late 1800s that ectomycorrhizal associations were first described as being either beneficial or is some cases even necessary for certain plants (Bonfante, 2018). It wasn’t until 1950 that we finally had experimental validation showing the benefits of a symbiotic relationship between ectomycorrhizal fungi associated with the roots of apple trees compared to those grown in sterile soil (Melin & Nilsson, 1950). This ignorance gap of the crucial roles that fungi play associating with a predicted 85% to even over 95% of plant species shows how much catching up we have to do (Lowenfels, 2017).
Plant and tree associations with mycorrhizal fungi have been shown to improve the efficiency of nutrient uptake of nutrients such as nitrogen, phosphorus, and many trace minerals. They have also been shown to improve water uptake, increase root surface area up to 100 fold and increase resistance to a myriad of pathogens (Lowenfels, 2017). It is funny how this experimental validation of fungi living in symbiosis with other plants in 1950 was discovered right around the same time as the green revolution when humans began to heavily rely on chemical fertilizers and pesticides. If this knowledge gap had been branched sooner, then maybe we could have better utilized these nutrients and reduced our needs for pesticides. Today, we may not be looking at the amount of pollution and energy usage that goes into producing and utilizing these fertilizers and pesticides. Fossil fuels are used to transport these inputs, often used for energy to produce them, and many are derived from petrochemicals themselves (Pimentel, 1971). Synthetic nitrogen fertilizer itself accounts for more than 50% of all the energy usage that goes into commercial agriculture (Woods et al., 2010).
In this paper, I would like to address the issues of how fungi can be used to improve our forestry practices, though many of the principles shared can be applied to agricultural, horticultural and even home gardening systems. I will introduce some basic concepts of fungal physiology so that one will be able to understand how to best apply these practices of utilizing fungi to promote ecological resilience within forested ecosystems. Methods for how to utilize and promote beneficial fungi in forestry systems will be detailed and resources shared so that foresters, private landowners and any person involved in reforestation efforts can easily implement these practices.
Fungal Physiology
It is important to distinguish the difference between a mushroom and a fungus. Mushrooms are the fruiting bodies of fungi. They are designed to help disperse spores which are used to help the fungus reproduce. The majority of fungi do not produce mushrooms however and have other reproductive structures such as conidiophores that they use for reproduction. The majority of the mushroom producing fungi belong to the order Basidiomycota.
Biochemical Digesters and Messengers
The fungus produces a network of threadlike structures called hyphae. Hyphae are very thin, only one cell wall thick. For reference, hyphae are about 4-6 micrometers thick and a single human hair is about 100 micrometers thick (Lowenfels, 2017). Hyphae can be seen as synonymous with the roots and root hairs of plants. They explore and sense their environment, growing towards areas where they sense nutrients, water, gases or signals from other organisms. They secrete enzymes for extracellular digestion. This means that they first digest their food outside of themselves in their surrounding environment, then suck in the digested food after. They store many of these enzymes inside their vacuoles as well as compounds for regulating the pH in the local environment. Hyphae also send out chemical messengers to communicate with plants and other microorganisms within the soil.
Fungi aid in the breakdown of nutrients that are normally locked up in the soil through freeing up these locked up nutrients with specialized enzymes. The plant by itself cannot break down many of the inorganic compounds which lock up these nutrients in the soil and the presence of fungi is required to allow the plant access to this nutrition. In exchange for the nutrients and water that the fungus provides the plant, the plant provides its fungal symbionts with photosynthates in the form of carbon sugars. A tree host may provide around 15% of its photosynthetically derived carbon sugars to its fungal symbionts, but in return receives around 85% of its nitrogen along with a myriad of trace elements and phosphorus (Lowenfels, 2017).
Fungal Structure
Some fungi may be unicellular and do not produce hyphae like yeasts, while others are multicellular. The hyphal fungi, like ourselves, are aerobic organisms that require oxygen to survive and exhale carbon dioxide. Their cell walls are mainly composed of chitin just like arthropods and insects. This is a major difference between fungi and plants, which have cell walls that are mainly composed of cellulose. Hyphae contain many organelles including the microfilaments and microtubules that help to transport nutrients like phosphorus magnesium and calcium.
Hyphae will mass together to create a thicker hyphal matrix below the soil. This collective mass of hyphae is known as mycelium. With mycorrhizal species of fungi, the mycelium creates networks that will form into symbiotic relationships, often with multiple plants of the same species, or even with multiple plants of different species.
Symbioses
The plants are the deciders in whether they form into a symbiotic relationship or not with a fungus. If a plant has excess nutrients, especially nitrogen and phosphorus, then it will not secrete certain hormone signals into the soil like strigolactones to attract potential symbionts. These specific hormone signals call out to nearby fungal spores and hyphae to let them know that they want to form into a symbiotic relationship with them. Plants also send out signals to potential mycorrhizal fungi when they are experiencing drought stress. Studies have shown that fungi can improve the plant’s access to water by 50 to 100 times more with the fungus creating a storage reservoir of water around the plant’s roots (Lowenfels, 2017). This is a case where less is more. When the plants are given fewer nutrients and less water, they are more likely to form into these symbiotic relationships where they can better utilize these inputs through their fungal symbionts.
Mycorrhizal fungi are more often than not obligate symbionts, meaning that without a host they will perish. Though spores can stay dormant in the soil until a host plant sends out a chemical messenger to wake it from its dormancy, their viability will decrease over time. That is why it is important to maintain mycorrhizae in the soil by keeping plant hosts available and avoiding long fallow periods.
Certain plants like those in the Brassicaceae or Amaranthaceae families do not form into mycorrhizal relationships or rarely do, and will not help to maintain symbiotic fungi in the soil (Glen et al., 1985). With roughly 95% of vascular plant species associating with fungi, these few plants are the exceptions and not the rule (Lowenfels, 2017). Since there are many weedy species in the Amaranthaceae and Brassicaceae such as pigweed (Amaranthus retroflexus) and Shepherd's purse (Capsella bursa-pastoris), using less water and adding less fertilizer will help to ensure that your plants confer a competitive advantage due to their enhanced access to these resources due to the nutrition gained by the symbiosis of their mycorrhizal fungi that these weedy species cannot access (Lowenfels, 2017).
Saprophytic Fungi
Saprophytic fungi play the crucial role of helping to decay dead material. Fungi are special in the fact that they have enzymes that can decompose complex materials which few other organisms can degrade such as lignin. Lignin is the organic polymer that gives plants structure and is highly abundant in woody species. These fungi return nutrients previously taken up by now dead organisms back into the soil so that new life can spring forth.
Figure 1. The saprophytic and occasionally parasitic fungus Hericium abietis, seen fruiting off decaying old growth douglas fir (Pseudotsuga menziesii).
Mycorrhizal fungi
Mycorrhizal fungi survive through making symbiotic relationships with other plant species. The fungus provides its host with nutrients and water. It is especially adept at providing its host with nitrogen and phosphorus. When mycorrhizal fungi associate with plants they can increase the amount of surface area access to soil water and nutrients up to 100 fold (Lowenfels, 2017). The 2 main forms of mycorrhizal fungi we will discuss are ectomycorrhizae (EM) and arbuscular mycorrhizae (AM).
Figure 2. The edible mushroom Amanita calyptroderma is an ectomycorrhizal species found here associating with douglas fir trees (Pseudotsuga menziesii)
Ectomycorrhizal Fungi (EM)
Ectomycorrhizal fungi form a protective sheath of hyphal threads covering the roots of their host plant. They create a structure called a Hartig net in the outer layer (apoplast) of the endodermis of the cells of the root (Figure 3.). The Hartig net is what allows for nutrient exchange between the fungus and its host. The majority of mycorrhizal species that form mushrooms are EM. This ability to make mushrooms as a mode of reproduction allows their spores to be spread through the air. Many of our choice edible species of mushrooms are EM (Figure 2.).
Figure 3. Hartig net shown formed outside the endodermis, hence the name ecto meaning outer (Piepenbring, 2015)
Arbuscular Mycorrhizae (AM)
Arbuscular mycorrhizae are endomycorrhizal, meaning that they penetrate directly into the cortical cells of the root. Inside these cortical root cells are where they form arbuscules, branch-like structures that aid in the nutrient exchange between them and their host (Figure 4). These species are much more likely to be associated with annual plants or with trees in warmer climates or more tropical regions with higher rates of decomposition (Figure 6). They produce ascospores under the soil which are very heavy unlike the many of the EM spores which can be distributed throughout the air after they are released from the fungal fruiting body. This means that AM spores must be within the vicinity of a viable host to come into a symbiotic relationship before their viability wears out.
Figure 4. Arbuscule inside the cortical cell of a root. Its tree-like branch structure is why the word arbuscular comes from the word arbor meaning tree (Vandegrift, 2011).
Fungal Forest Ecology
Fungi play an integral role in maintaining healthy forest ecosystems. When they form into a symbiotic relationship with trees and plants, they drastically improve the nutrient and water scavenging abilities of their hosts. They even allow for the exchange of photosynthates of the taller more mature trees who receive the majority of sunlight from the top of the canopy, to share their harvested light in the form of photosynthate carbon sugars with their progeny growing in the shade beneath the forest canopy (Stamets, 2005). They accomplish this feat through their shared mycorrhizal network (Teste et al., 2008). A study from the University of British Columbia found that the false truffle (Rhizopogon sp.) is one of the fungi responsible for the distribution of nutrients from a dying tree, not only to its offspring but to other species as well (Frazer, 2015). The most well-connected tree they found was connected to 47 other trees through the mycelial network of 8 individual Rhizopogon of the same species (Frazer, 2015). A single individual Rhizopogon was even shown to associate with 19 different trees both young and old (Frazer, 2015). It has been shown that understory seedlings of Douglas Fir (Pseudotsuga menziesii) received 21% of their water from mature trees through their associated mycorrhizal network (Schoonmaker et al., 2010).
This ensures that the next generation of trees will have the nutrition needed once it is time to grow their way to the top of the canopy once their elders eventually pass on. That is why it is important to maintain older “Hub” trees in forest reestablishment, so that they may share their mycorrhizal networks with the younger seedling trees (Simmard, 2009). Communication networks have been observed between trees through their shared mycorrhizae. Chemical messengers can be transported through the mycelial network to alert other trees of potential pathogen pressures (Simmard, 2018). This mycorrhizal messaging system has led to enhanced understory seedling survival, growth, nutrition, and mycorrhization, increased defense chemistry and kin selection, and demonstrates collective memory-based interactions among trees and fungi (Simmard, 2018). This exemplifies a mutualistic view of the forest ecosystem where the older generations work to feed the younger generations. All made possible through the help of these intricately interwoven mycelial networks.
Once these older trees eventually die, the saprophytic fungi work to decompose these giants back into the soil from which they grew, freeing up the nutrients locked within their decaying forms to allow new life to spring forth. Fungi have the amazing ability to decay lignin, the complex organic polymer which composes the major structural elements of trees. Lignin is very hard to decompose and it has taken millions of years for fungi to develop the ability to create enzymes that can degrade lignin. Lignin degrading organisms were rare and nearly absent during the Paleozoic period, but early fungi of the order Polyporales were thought to be some of the first to evolve the lignin peroxidases that lead to a decrease in coal during the Carboniferous era (Ayuso-Fernandez et al., 2018). This evolution is a major factor in why coal will never be a renewable resource since we now have so many fungi, especially the white-rot fungi, which are adapted to degrading this organic polymer that would otherwise form into coal over millions of years (Ayuso-Fernandez et al., 2018). Lignin composes roughly 25% of the tree’s mass (Novaes et al., 2010). Imagine a world where fungi could not decompose lignin and a quarter of the mass of all trees didn’t degrade. Considering that trees have been on this planet for roughly 400 million years, that’s a lot of biomass leftover on the earth's surface. We can thank fungi for their ability to break lignin down back into the soil so that today we are not currently wading through an obscene amount of undegraded lignin and have fertile soil instead.
Pathogen Protection
Mycorrhizal fungi provide extra protection for their hosts from pathogens such as nematodes, bacteria, and other fungi. The sheath that ectomycorrhizae form around the roots of many tree species keep a protective layer around these roots. Many mycorrhizal fungi are capable of secreting enzymes that may degrade potential pathogens and can secrete many antimicrobial compounds (Nematode Trapping Fungi, 2013). Many AM fungi even make snare traps to capture nematodes (Figure 5). The association of endophytic fungi with tree and plant species has also been shown to increase the quantity of protective secondary metabolites produced by the host and even in some cases, the secondary metabolites are produced by the fungus itself (Lowenfels, 2017). Many of these secondary metabolites are used specifically for plant host defense and innate immunity.
Figure 5. Some species of fungi create snare traps, release nema-toxic compounds or are endoparasites of nematodes (Nematode Trapping Fungi, 2013)
Fungal Forestry For Climate Resilience
The major cause of climate change has come from the drastic alteration of our terrestrial hydrologic cycles caused by deforestation. Though carbon dioxide does play a large role in climate change, the interplay between the pressure and moisture differentials of our forests, with that of the insulating forces of our oceans and other large bodies of water is what largely drives our weather patterns. Forests affect climate by creating the low-pressure zones over which higher pressure warm air currents created by the insulating forces of our oceans are drawn onto land (Makareiva et al., 2014).
The release of polar nuclei in the form of spores and pollen also aids in regulating terrestrial hydrologic function. This polarity of spores is due to the fact that they have extremely hydrophilic (water-loving) compounds like mannitol on their surface. This is why they are thought to aid in the formation of rain clouds (Hasset et al., 2015). Spores and pollen released into the air above our forests congeal with water as the warm humid air over our oceans sinks into the lower pressure zones created by our forests, causing clouds to form and rain to fall (Sheil et al., 2009). This is how forests help to maintain the natural hydrologic functions of our planet and keep water returning to the land.
Considering that we have lost 46% of our world's trees since we first began harvesting them (Nunez, 2019), it is no wonder that we are now experiencing the drastic shifts in climate that we are today. Reforestation with the added benefits of incorporating mycorrhizal fungi will be key in reversing the effects of climate change. Fungi also add the benefit of storing carbon deep in the soil in the form of the carbon-rich glycoprotein glomalin, rather than having that carbon decompose on the surface just to be returned to the atmosphere as CO2. In the boreal forests of Sweden, they even found that the majority of the forests carbon, 50%-70% was located deep in the soil, derived from roots and root-associated microorganisms. They found that fungi were essential in regulating the carbon storage capabilities of these forest ecosystems (Clemmensen et al., 2013). This is surprising since you would expect the majority of organic carbon would be dropped on the surface of the soil in the form of needles and leaf litter (Wang et al., 2017).
Fungal carbon stores like glomalin, improve soil water holding capacity, create a healthy soil structure that improves the soil habitat for other microorganisms. Glomalin may stay intact in the soil for up to 4 decades and gives soil that characteristically healthy sticky structure (Lowenfels, 2017). Many of our AM fungi, especially those in the order Glomeromycota are responsible for putting glomalin in the soil. Glomalin accounts for 27%-30% of the carbon stored in the soil when mycorrhizal fungi are present (Lowenfels, 2017).
Clearly, mycorrhizal fungi offer a myriad of benefits to forest ecosystems and are integral for their survival. We can improve forestry practices by including mycorrhizal fungi when we plant trees. It is important that we use the proper mycorrhizal symbionts if we are to use them for forest replants. Though many species of trees and plants, as well as their fungal symbionts, can be quite generalist when it comes to who they form a symbiotic relationship with, there are also some that are quite specific in what species they will form a relationship with. A good place to start is determining whether the plant or tree species you are working with is more likely to form an arbuscular or ectomycorrhizal relationship with a fungus. A general rule of thumb seems to be that regions with rapid rates of decomposition due to high temperatures and high amounts of precipitation like tropical forested areas seem to have tree species associated mainly with AM. The opposite seems to be true for EM, especially in northern latitudes that experience slower rates of decomposition due to lower temperatures and less precipitation (Figure 6.). This seems to ring true for elevation as well where higher elevations seem to have higher rates of EM than AM (Steidinger et al., 2019). Though EM only accounts for roughly 2% of mycorrhizal species, they clearly play a huge role in creating symbiotic relationships with the majority of northern temperate and high elevation tree species (Steidinger et al., 2019). It is not uncommon for a tree to begin its life associated with AM and then switch to EM later on in life. This is especially true with trees in the Pinaceae family (Lowenfels, 2017).
Figure 6. World map showing the predicted percentage of tree basal area and distribution of ectomycorrhizal (EM) and arbuscular mycorrhizal (AM) species. Noting a higher concentration of AM in tropical high temperature, high rainfall areas, and higher concentrations of EM in more temperate northern regions with lower temperatures and rainfall. This association is thought to be related to rates of decomposition (Steidinger et al., 2019).
Applications of Mycorrhizal Forestry
There is a lot of research out there on mycorrhizal species that are associated with various tree species. A search on Google Scholar can help to reveal what species you may want to target with your mycorrhizal products when doing a forest replant. The book Teaming With Fungi is also a great resource for finding the correct fungal symbiont for the species you are working with. If you cannot locate any research on the particular fungal symbiont that works with your tree species, then using the above map may help you decide if you are more likely to need EM or AM. Using species of EM or AM that are more generalist in nature can help to ensure that a symbiotic relationship is formed between them and your tree species. Keep in mind that a tree may require different symbionts as a seedling then it will when it matures. Many tree species may associate with multiple mycorrhizal species that can be EM, AM or both.
Mycorrhizal Inoculants
There are various mycorrhizal inoculant products out there on the market that come in different forms from powders, to liquids, or added into other products like compost. Many contain spores or hyphal fragments. The labels of these products will indicate which species are contained within the mixture and the above information can help you to decide whether these particular fungi will be useful for your specific project. It is recommended to follow the instructions that these products come with though there are a few rules of thumb that seem to be ubiquitous. First, it is important to make sure the inoculant makes close contact with the roots. The closer the inoculum is to the roots, the more likely it will be able to effectively associate with them. Sprinkling spores onto germinating seeds is a great way to get that symbiosis started early and give them a head start in life. Another important consideration is to make sure you use your inoculum in a timely manner. Many spores can stay viable for a year but their viability will start to drop significantly so it is recommended to use most products within a year and not much longer than 2 years.
Utilizing Indigenous Mycorrhizae
Using indigenous mycorrhizal species is often the best course of action. Often nurseries that produce trees for replant use sterilized soil to avoid pathogen contamination. This can be detrimental though since it deprives the young trees of forming into a beneficial symbiotic relationship with fungi and other microbes early on in life. In the end, the tree will be planted out in nature where the soil is not sterilized, so ensuring that you have trees that are resilient against potential pathogens and have already formed relationships with the indigenous microorganisms like fungi from where they will eventually be planted out can help to ensure that our seedling trees have enough vitality to survive. This can be done by inoculating the nursery soil with the indigenous microorganisms prior to transplanting. Incorporating native soil that is rich in indigenous microorganisms is an easy way to accomplish this. It has been shown that transplanting using soil growing close to the species that you are transplanting can significantly increase its growth (Peay, 2018). The competitive advantage that these mycorrhizal fungi confer is greatly increased the sooner that they are applied (Peay, 2018). Adding biochar, and increasing organic matter and carbon in the soil can help these beneficial fungi and other microorganisms to proliferate.
The simplest way of getting your seedlings inoculated with beneficial indigenous mycorrhizae is to plant them close to mature trees, especially those of the same species (Grove et al., 2019). This means that focusing reforestation efforts on the edges of current forests can help aid in increasing tree seedling resilience. This makes sense since in nature you would expect seeds to end up within close proximity to the parent trees or trees of the same species which already have a robust network of mycorrhizal fungi from which the growing seedling can associate with. That is why there is often a higher mortality rate of seedlings planted more towards the middle of clearcut than those planted along edge forest habitats (Grove et al., 2019).
Most EM fungi live in the highest abundance within the top 20 cm of soil (Wallander et al., 2004). Therefore compaction and displacement of topsoil from clear cuts can greatly disturb the indigenous EM fungi. The loss of tree canopy will increase irradiance of the soil, increasing soil evaporation and temperature leading to a further decrease in EM fungi (Fernandez et al., 2017). Once the host trees are removed, many of the mycorrhizal fungi will lose their carbon source and may likely perish unless there are nearby hosts from which to form into a new symbiotic relationship with (Jones et al., 2018).
If an invasive plant that associates with different mycorrhizal species ends up colonizing the area first, then you are likely to see a decrease in the mycorrhizal fungi that will be desirable hosts for the replanted trees. This host specificity of mycorrhizal fungi can help to confer a competitive advantage to your replanted trees if there's an abundance of mycorrhizae in the soil that will associate with your desired species that outnumber mycorrhizae that may associate with invasive or weedy species. Heavy soil disturbance and decreases in forest edge habitat can decrease this competitive advantage conferred by the indigenous mycorrhizal fungi.
Wet Sieve Method
Getting higher concentrations of indigenous mycorrhizae can be accomplished through techniques such as wet sieving where the soil with desired fungal species is sieved through a series of mesh screens down to a final 50-micrometer sieve. This final sifted material can then be flotation separated using water mixed with glycerol or sucrose. It is best to sieve material from or nearby where the seedlings will be planted out. Collecting soil for sieving near older trees of the same species as your seedlings will ensure that the soil contains fungal hosts specific to your seedlings. A great resource guide for this method can be found in the book Teaming With Fungi (Lowenfels, 2017) and in the online pdf. at (Pacioni, 1991).
Activated Biochar
Biochar is created by burning down plant matter or manure in an oxygen-free or a low oxygen environment. The resulting charcoal is covered with many pores of various sizes and in turn, has a tremendous amount of surface area. These pores create ideal habitats for a huge variety of beneficial microorganisms including many fungi, including glomalin producing AM fungi of the Glomeromycota, and the nematophagous Trichoderma fungi (Batista, 2018). Biochar applied in forests has been shown to increase soil porosity, soil moisture retention, and aggregate stability while reducing soil bulk density (Li et al., 2018). It also increases the amount of soil carbon storage, tends to increase cation exchange capacity and increases concentrations of available phosphorus and potassium (Li et al., 2018).
Biochar acts as a long term storage mechanism for carbon in the soil. Biochar is a stable carbon form that can last in the soil for thousands of years (Rawat et al., 2019). According to a study at Cornell University, if we added biochar to 10% of our global croplands, we could sequester the equivalent of 29 billion lbs of carbon dioxide (Hertsguard, 2014). Al Shay, senior instructor in the Horticulture department at Oregon State University and a veteran of the landscaping industry for over 30 years has spent many years gathering empirical evidence for the benefits of incorporating mycorrhizal fungi and biochar when planting trees. Simply planting some trees with these inputs and others without and seeing how they have grown over the years has him convinced of the potential these inputs have in increasing the resilience and vigor of trees. A recent meta-analysis of biochar use in forestry showed up to a 41% increase in biomass, especially in younger seedlings (Thomas & Gale, 2015).
It is important to note that not all biochar is created equally. With a huge variance in what can be used as biochar from all forms of manure and plant materials, some materials may work better than others. One example would be that they found that chicken manure compared to all other manure biochars, was the superior biochar for cleaning up mercury from power plants (Hertsguard, 2014).
It is also important to “activate” your biochar before adding it to soils. By activate, I mean inoculating the biochar with microbes before adding it to the soil or else it has the potential to keep water and nutrients away from plants due to the hygroscopic pressure from the adhesive tension created by its numerous micropores. Luckily it is quite easy to activate biochar. Simply add a small amount of bokashi to your biochar or put biochar into your compost at least 6 weeks before adding it into the soil during transplanting. A small handful of biochar, compost, and mycorrhizal fungi inoculant to the roots of your transplant will ensure that they are fully established with a diversity of beneficial microorganisms with plenty of habitats for years to come.
Conclusion
Looking at an uncertain future with the dangers associated with climate change and pollution from excess fertilizer usage and excess energy usage, it is now more important than ever that we put our efforts into reforestation. By utilizing mycorrhizal fungi into our reforestation efforts, we can help to ensure that our trees and plants will be more resistant to drought, pest pressures and have greater access to nutrients. In some cases, especially with EM and saprophytes, we get the added benefit of a wild mushroom food and medicine source.
By utilizing techniques such as inoculating our seeds and seedling with the native soil containing the indigenous fungi and microorganisms, adding activated biochar, or using the wet sieve method to harvest indigenous spores, we can ensure that our reforestation efforts will have the added resilience conferred by these symbionts necessary to protect these forests and give them vigor in an uncertain future. The carbon storage and properly functioning terrestrial hydrologic cycles created by healthy forests full of fungi will be our greatest tools in combating climate change. The more we promote diversity and allow the complexity of these organisms to flourish, the greater benefits we will receive at a time when climatic resilience will determine our future.
Citations
Ayuso-Fernández, I., Ruiz-Dueñas, F. J., & Martínez, A. T. (2018). Evolutionary convergence in lignin-degrading enzymes. Proceedings of the National Academy of Sciences of the United States of America, 115(25), 6428–6433. https://doi.org/10.1073/pnas.1802555115
Batista, E. M., Shultz, J., Matos, T. T., Fornari, M. R., Ferreira, T. M., Szpoganicz, B., ... & Mangrich, A. S. (2018). Effect of surface and porosity of biochar on water holding capacity aiming indirectly at preservation of the Amazon biome. Scientific reports, 8(1), 1-9.
Bonfante, P. (2018). The future has roots in the past: the ideas and scientists that shaped mycorrhizal research. New Phytologist, 220(4), 982-995
Clemmensen, K. E., Bahr, A., Ovaskainen, O., Dahlberg, A., Ekblad, A., Wallander, H., ... & Lindahl, B. D. (2013). Roots and associated fungi drive long-term carbon sequestration in boreal forest. Science, 339(6127), 1615-1618.
Desprez-Loustau, M. L., Marçais, B., Nageleisen, L. M., Piou, D., & Vannini, A. (2006). Interactive effects of drought and pathogens in forest trees. Annals of forest science, 63(6), 597-612.
Fernandez, C. W., N. H. Nguyen, A. Stefanski, Y. Han, S. E. Hobbie, R. A. Montgomery, P. B. Reich, and P. G. Kennedy. 2017. Ectomycorrhizal fungal response to warming is linked to poor host performance at the boreal‐temperate ecotone. Global Change Biology 23:1598–1609.
Fu, Y. H., Zhao, H., Piao, S., Peaucelle, M., Peng, S., Zhou, G., ... & Song, Y. (2015). Declining global warming effects on the phenology of spring leaf unfolding. Nature, 526(7571), 104-107.
Glenn, M. G., Chew, F. S., & Williams, P. H. (1985). Hyphal penetration of Brassica (Cruciferae) roots by a vesicular–arbuscular mycorrhizal fungus. New phytologist, 99(3), 463-472.
Goodess, C. M., Palutikof, J. P., & Davies, T. D. (1992). The nature and causes of climate change: assessing the long-term future. Belhaven Press.
Gorte, R. W., & Sheikh, P. A. (2010). Deforestation and climate change.
Guignard, M. S., Leitch, A. R., Acquisti, C., Eizaguirre, C., Elser, J. J., Hessen, D. O., ... & Soltis, D. E. (2017). Impacts of nitrogen and phosphorus: from genomes to natural ecosystems and agriculture. Frontiers in Ecology and Evolution, 5, 70.
Hassett, M. O., Fischer, M. W., & Money, N. P. (2015). Mushrooms as rainmakers: how spores act as nuclei for raindrops. PloS one, 10(10).
Jones, M. D., D. M. Durall, and J. W. G. Cairney. 2003. Ectomycorrhizal fungal communities in young forest stands regenerating after clearcut logging. New Phytologist 157:399–422.
Makarieva, A. M., Gorshkov, V. G., Sheil, D., Nobre, A. D., Bunyard, P., & Li, B. L. (2014). Why does air passage over forest yield more rain? Examining the coupling between rainfall, pressure, and atmospheric moisture content. Journal of Hydrometeorology, 15(1), 411-426.
Melin E, Nilsson H. 1950. Transfer of radioactive phosphorus to pine seedlings by means of mycorrhizal hyphae. Physiologia Plantarum 3: 88–92.
Murray, J., & Dey, C. (2009). The carbon neutral free for all. International journal of greenhouse gas control, 3(2), 237-248.
“Nematode Trapping Fungi.” Microbewiki, MediaWiki, 22 Apr. 2013, https://microbewiki.kenyon.edu/index.php/Nematode_trapping_fungi.
Ng JMS, Han M, Beatty PH, Good A (2016) Genes, meet gases: the role of plant nutrition and genomics in addressing greenhouse gas emissions. In: Edwards D, Batley J (eds) in Plant Genomics and Climate Change. Springer, New York, NY, pp 149–172
North, M. P., Stevens, J. T., Greene, D. F., Coppoletta, M., Knapp, E. E., Latimer, A. M., ... & Young, D. J. (2019). Tamm Review: Reforestation for resilience in dry western US forests. Forest ecology and management, 432, 209-224.
Novaes, E., Kirst, M., Chiang, V., Winter-Sederoff, H., & Sederoff, R. (2010). Lignin and biomass: a negative correlation for wood formation and lignin content in trees. Plant Physiology, 154(2), 555-561.
Nunez, C. (2019, February 25). Deforestation and Its Effect on the Planet. Retrieved from https://www.nationalgeographic.com/environment/global-warming/deforestation/
Pimentel, D. (1971). Ecological effects of pesticides on non-target species. Office of Science and Technology.
Li, Y., Hu, S., Chen, J., Müller, K., Li, Y., Fu, W., ... & Wang, H. (2018). Effects of biochar application in forest ecosystems on soil properties and greenhouse gas emissions: a review. Journal of soils and sediments, 18(2), 546-563.
Lowenfels, J. (2017). Teaming with Fungi: the organic growers guide to mycorrhizae. Portland, OR: Timber Press.
Pacioni, Giovanni. (1991). Wet-sieving and decanting technique for spores extraction.
Steidinger, B. S., Crowther, T. W., Liang, J., Van Nuland, M. E., Werner, G. D., Reich, P. B., ... & Herault, B. (2019). Climatic controls of decomposition drive the global biogeography of forest-tree symbioses. Nature, 569(7756), 404-408.
Sheil, Douglas, and Daniel Murdiyarso. “How Forests Attract Rain: An Examination of a New Hypothesis.” OUP Academic, Oxford University Press, 1 Apr. 2009, https://academic.oup.com/bioscience/article/59/4/341/346941.
Simard, S. W. (2009). The foundational role of mycorrhizal networks in self-organization of interior Douglas-fir forests. Forest Ecology and Management, 258, S95-S107.
Simard, S. W. (2018). Mycorrhizal networks facilitate tree communication, learning, and memory. In Memory and learning in plants (pp. 191-213). Springer, Cham.
Stamets, P. (2005). Mycelium running: how mushrooms can help save the world. Berkeley, CA: Ten Speed Press.
Staples, T. L., Mayfield, M. M., England, J. R., & Dwyer, J. M. (2019). Comparing the recovery of richness, structure, and biomass in naturally regrowing and planted reforestation. Restoration Ecology.
Teste, F. P., & Simard, S. W. (2008). Mycorrhizal networks and distance from mature trees alter patterns of competition and facilitation in dry Douglas-fir forests. Oecologia, 158(2), 193-203.
Vandegrift, Roo. “ArbuscularMycorrhizae.” Flickr, Yahoo!, 29 Aug. 2011, https://www.flickr.com/photos/werdnus_roo/6091498087.
Wallander, H., H. Goransson, and U. Rosengren. 2004. Production, standing biomass and natural abundance of N‐15 and C‐13 in ectomycorrhizal mycelia collected at different soil depths in two forest types. Oecologia 139:89–97.
Wang, W., Zhong, Z., Wang, Q., Wang, H., Fu, Y., & He, X. (2017). Glomalin contributed more to carbon, nutrients in deeper soils, and differently associated with climates and soil properties in vertical profiles. Scientific reports, 7(1), 1-13.
Ward, M. H. (2009). Too much of a good thing? Nitrate from nitrogen fertilizers and cancer. Reviews on environmental health, 24(4), 357-363.
Woods, J., Williams, A., Hughes, J. K., Black, M., & Murphy, R. (2010). Energy and the food system. Philosophical Transactions of the Royal Society B: Biological Sciences, 365(1554), 2991-3006.
Comentários